UA Physics Explores Superfluidity in 2-D!
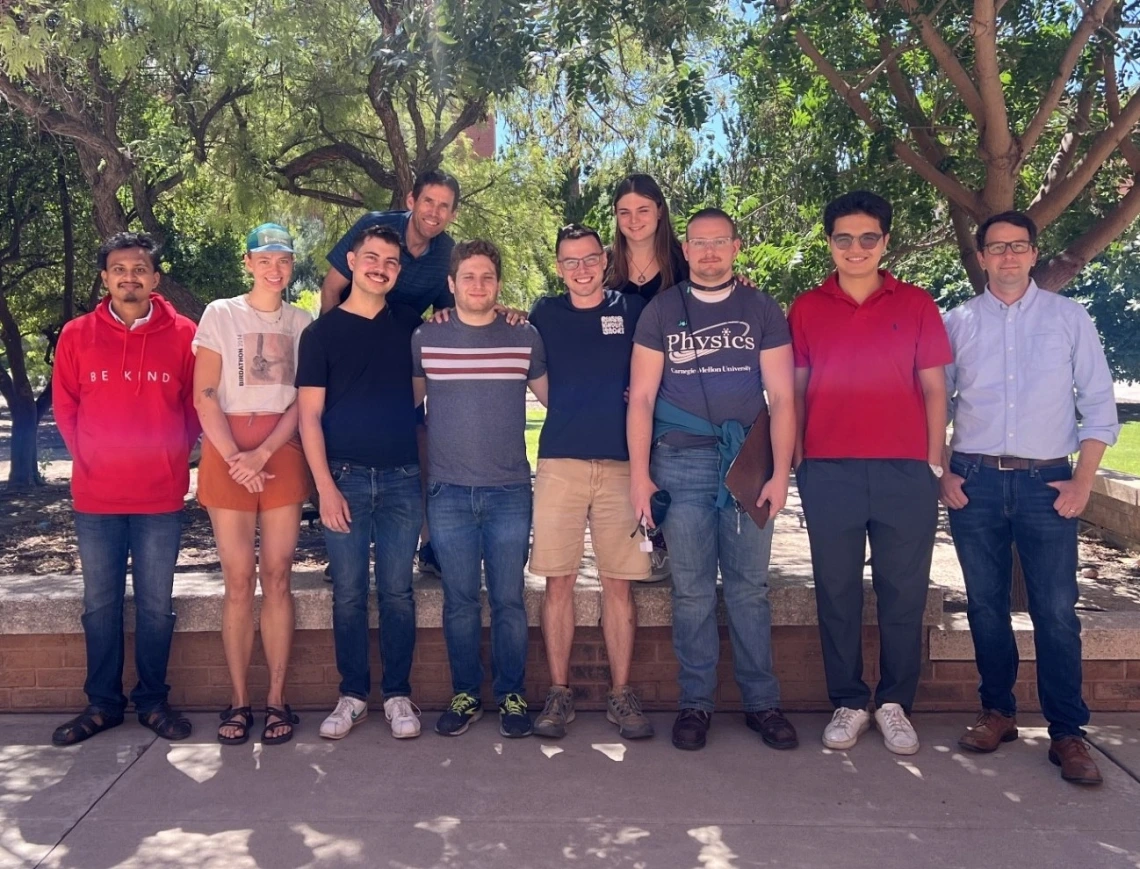
Superfluids are liquids without viscosity; that is, they flow without losing any kinetic energy. Superfluid states of matter offer the possibility of transporting energy and information without dissipation, which can be used to make high-speed and low-energy electronics.
The research group of Prof. John Schaibley and Prof Brian Leroy, which includes students Jacob Cutshall, Fateme Mahdikhany, Anna Roche, and Daniel Shanks, has been making important advances in the field of 2D-superfluidity. In a paper recently published in the journal Science Advances the group was able to demonstrate superfluidity in an atomically thin semiconductor device.
Condensed matter physics in 2D poses unique challenges, as, unlike in 3D, it is impossible to create true long-range order in 2-dimensions. Long-range order refers to how elements in a system correlate with one another and has important implications for the creation of large lattice structures. The lack of true long-range order in 2D systems makes it challenging, if not impossible, to create the conditions needed to facilitate Bose-Einstein condensation in a 2D system. However, The Berezinskii-Kosterlitz-Thouless (BKT) transition allows for the observation of quasi-long-range ordered superfluids in 2D. Using BKT physics, Schaibley's group approximated long-range ordering to create a 2D structure with superfluid properties.
To do this, Schaibley's group stacked layers of 2-dimensional materials into structures with distinct properties and functionalities not found in standard materials. These structures are called Van der Waals heterostructures and are considered extremely important in the development of new electronic technologies. 2D heterostructures with superfluid properties have been claimed to have been achieved by other research groups, but the Schaibley group was the first to create such a structure that could be verified to have superfluid properties by direct optical measurement, the first direct observation of its kind. Moreover, the group was able to map the phase change of the superfluid and found that it maintained superfluidity up to a temperature of 15K, which was in agreement with theoretical prediction.
Schaibley's group's research is an essential step toward developing on-chip superfluid structures. Such structures would be invaluable to the study of fundamental physical behaviors and could be the key to quantum devices that use superfluidity.